Just like in our visible world, microorganisms exhibit various kinds of survival mechanisms. Of the many, biofilms stand out with their complex and yet to fully understand entity. Biofilms are complex dynamic, slime-like layers composed of a mix of microorganisms including organic substances like proteins, lipids embedded in the biofilm matrix. Biofilms confer a broad range of advantages to its members such as adhesion, nutritional sources, cellular communication, and protection from external stress. Also the biofilm matrix protects embedded microorganisms from antibiotics, disinfectants, or the host immune system reach. Biofilms are uninvited guests on many surfaces, ranging from sewage system pipes to your own mouth, where they cause tooth decay. They are also notoriously known to take over medical equipment, often reoccurring even after being subjected to antimicrobial agents.
Classic antimicrobial therapy often proves inadequate because it typically targets actively dividing cells, rather than persister cells, which are dormant cells residing within biofilms. These persister cells survive in a state of reduced metabolic activity. Additionally, biofilms are sometimes formed by drug-resistant bacterial species, presenting another challenge even when the bacteria become active. Furthermore, not all antibiotics can penetrate biofilms to effectively reach and eliminate the bacteria within. The complexities surrounding the eradication of biofilms from diverse surfaces and the human body have spurred the exploration of alternative therapeutic modalities. Among these options, bacteriophages and their other derivative products have emerged as promising candidates, either employed independently or in synergy with antibiotics and other agents which we will discuss in this article.
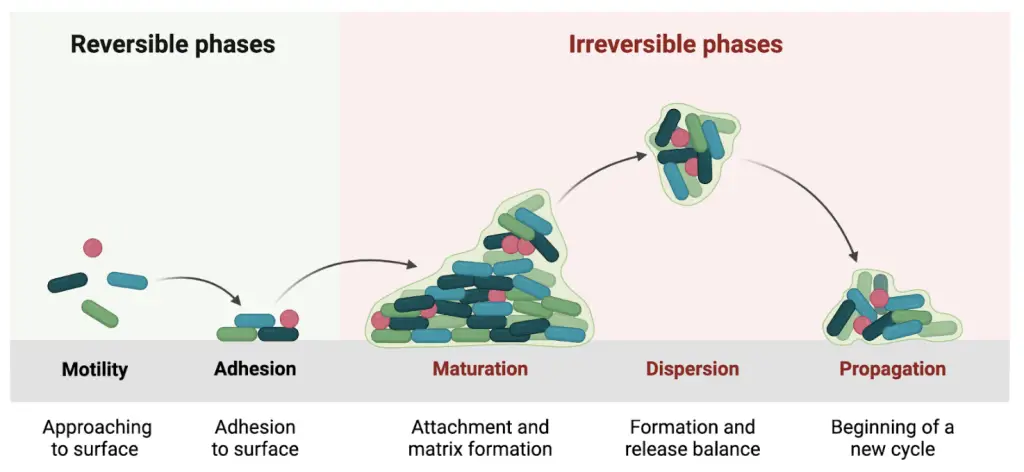
The biofilm formation takes place in various stages. The initial contact with a surface, irreversible contact with a surface, formation of microcolonies—expansion, maturation of the biofilm, and cell detachment of the individual cells from the matrix. Cellular structures, such as fimbriae and flagella, bacterial proteins—adhesins, and physical forces, are responsible for the colonization. The final phase of biofilm life occurs when the ratio of newly formed cells equals the number of dead ones. Environmental conditions such as oxygen depletion and nutrient unavailability result in the switching of bacterial metabolism. Enzymes (e.g.hydrolases and endonucleases) that break down the extracellular matrix, allowing individual bacteria to be released into the environment, are produced.
Occurrence of biofilms
Polymicrobial biofilm occurs in many natural environments, e.g., the oral cavity, where many microorganisms form dental plaques, intestines, or vaginas. Certain multi-species biofilm-related diseases can arise when a single pathogen is introduced into an existing microbiome, leading to dysbiosis or when opportunistic pathogens become virulent due to environmental imbalances. Other situations occur when one pathogen first adheres to the infection site as first and prepares the environment for another. Due to the recruitment of a new species, the gene pool is broadened, and it helps control and regulate the survival mechanisms of individual members.
Biofilm-associated polymicrobial communities are responsible for many diseases, e.g., bone infections and osteomyelitis, gallbladder disease, various chronic middle-ear disease processes, chronic rhinosinusitis, chronic infections of the urogenital systems, e.g., bacterial vaginosis, dental infections, tonsillitis, surgical site infections, chronic non-healing wounds such as venous and diabetic ulcers, pressure sores, and burn injuries, respiratory infections, e.g., cystic fibrosis and medical device-related infection.
Using Phages against biofilms
The rate at which bacteria acquire antibiotic resistance is alarming and the current epidemiological situation requires the search for alternative methods of combating bacterial infections. One of the options is to use bacteriophages. Bacteriophages show high specificity, recognizing their host at the species and even strain level due to the presence of characteristic receptors on the surface of the bacterial cell.
The usage of phages has many advantages, e.g., rapid clearance from organisms, self-propagation in the site of infection, host specificity, opportunity to make a genetic modification, easy isolation, stability, and relatively low-cost production. The right phage must be selected carefully because not all have good therapeutic results. When choosing phages, some rules should be followed: specificity to target bacteria, lytic activity, and the lack of genes encoding bacterial virulence factors, antibiotic resistance products, and toxins. Only fully sequenced bacteriophages can be used for treatment in medicine.
Another concern about phage therapy is to optimize the dosage of virions and the method of administration to provide good delivery to the site of infection. After killing all pathogenic bacteria, the phages are removed from the body as they cannot multiply in eukaryotic cells. In addition, if selected carefully, phages are safe for the human microbiome.
On the other hand, they may be neutralized by the host’s immune system, removed from the body too quickly, and bring no profit. Another issue from the immune system might be an allergic reaction that may limit the scope of possible use of bacteriophages. Unfortunately, bacteria have developed multiple resistance mechanisms to phages (e.g., modification and blockage phage receptors on the bacterial surface), and even though phages have an equally impressive assortment of tools to overcome this resistance, it is better to use a cocktail of phages.
Using of phage extracts as a treatment against biofilms
Next, to phages themselves, lytic enzymes that they produce are also considered treatment factors. Bacteriophages synthesize enzymes such as peptidoglycan hydrolases, holins, and endolysins, which allow the release of progeny virions by destroying bacterial cell walls. Endolysin can induce lysis from within, as an antimicrobial agent active against pathogens. This refers mainly to Gram-negative bacteria, which need to be treated with additional factors. Other phage enzymes—depolymerase, are tail-spike proteins with the enzymatic domain or occurring as free molecules.
Phage-delivered enzymes are usually unique and species-specific. However, sometimes enzymes can show activity across a broad spectrum including strains and species. Treatment of polymicrobial communities usually requires several different enzymes or combined therapy. Bacteria rarely evolve resistance to lysins because they attack sites on the peptidoglycan cell wall critical to bacterial viability. Nevertheless, combining phage lysins and antibiotics, phages and other agents, or the production of genetically engineered enzymes may be more effective in infection elimination.
Lytic phage can be an effective weapon in the fight against biofilm, both in the context of preventing its formation and its eradication. The activity of phages in mature biofilm depends on the bacteria’s growth phase, placement, coaggregation with other cells, nutrient availability, access to receptors, and diffusion capacity. Phages can be used not only in biofilms of multi-bacteria species but also in bacteria–yeast ones. Phages can stimulate the host bacteria to produce EPS-degrading enzymes and proteases that degrade bacterial capsules. These actions collectively enhance the effectiveness of phages in combating biofilms.
Another approach is combining phages with nanoparticles. This solution may enhance phage penetration through biofilm. Moreover, nanoparticle migration in matrices might be modulated by a magnetic field in ex vivo models. In addition to antibiotics and nanoparticles, other chemical compounds or groups of compounds might be combined with phages to minimize formation and eradicate polymicrobial biofilm.
Phage-delivered enzymes are the next option to eliminate polymicrobial biofilm. Their main advantages are host specificity and easy matrix penetration and removal.
All these methods allow for better penetration of the biofilm matrix and reaching the surface of the target bacterial strains. The use of phage therapy is also increasingly used in medicine in the treatment of severe multi-species infections. The attempts to use phages as prevention factors include coating urinary catheters and disinfectants in hospital or industry environments is also being implemented. However, the routine use of bacteriophages in medicine still requires a lot of research, including optimization and legislative work. However, despite further work required, bacteriophages and therapies using them to any extent are the future in treating bacterial infections.
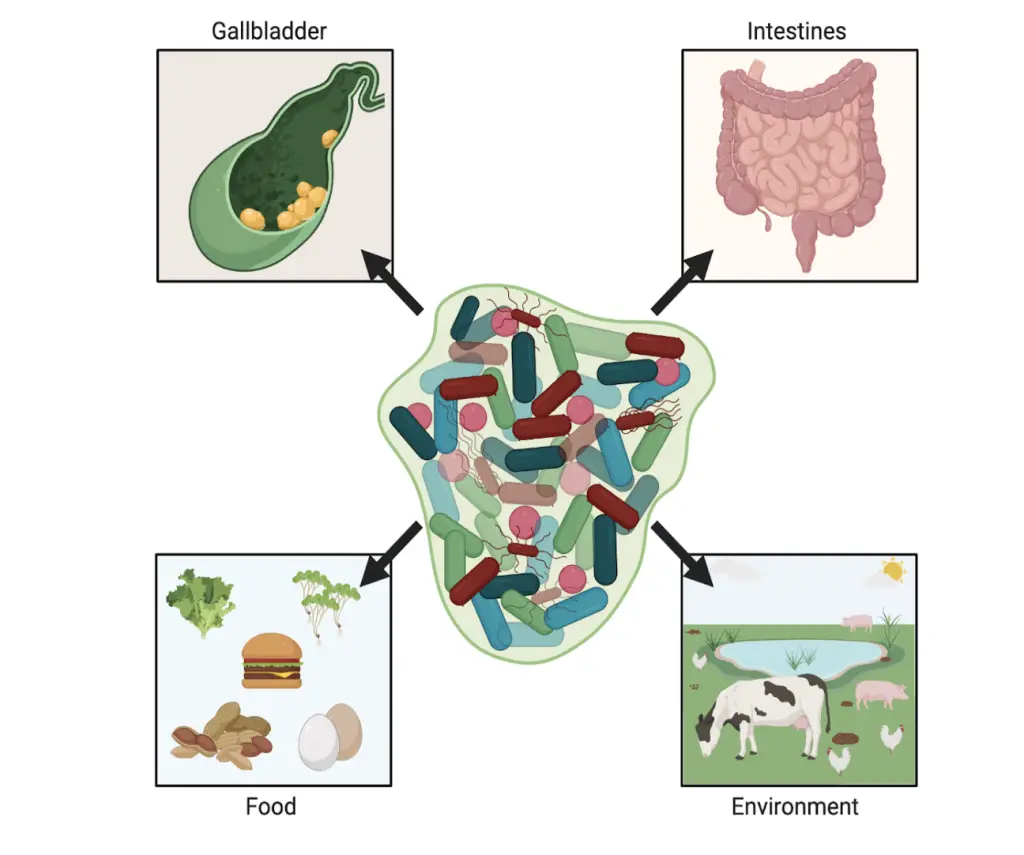
These viruses are and will be increasingly used to prevent bacteria in the hospital environment and other cases, e.g., in the food industry, veterinary medicine, or agriculture.
Bacteria Vs Phages
Bacteria can evolve to be insensitive to phage by changing their phenotypes in response to heterogeneous environments. Biofilm matrix comprises many bacterial enzymes, e.g., amidases and peptidases, that may inactivate phages. Moreover, in deeper biofilm layers, more dead cells occur, and phages may adsorb to them without any benefits for therapy. Molecules can also catch virions in the matrix. One of the ways of bacterial defense is the production of systems that interfere with phage nucleic acids, e.g., clustered regularly interspaced short palindromic repeats (CRISPR)–Cas9. Bacteria may also prevent phage DNA integration by a superinfection exclusion system or use an abortive infection system to block the synthesis of phage particle compounds. Another protection is related to hiding binding phage receptors by the production of curli polymer (CsgA) as extracellular fibers that curtain bacterial cells.
Enhancing phage performance in Biofilm
To intensify the action of phages, they can also be used with various groups of antibiotics (phage-antibiotic synergy (PAS) phenomenon). However, not every drug acts synergistically with selected phages and every combination should be checked in in vitro studies. Another mode of action of phages is interfering with bacterial efflux pumps, increasing the sensitivity to various drugs. Bacteriophages can also be combined with disinfectants such as chloride, hypochlorite, or quaternary ammonium compounds and enzymes, e.g., polysaccharides depolymerases. In addition, in the case of a biofilm with a heterogeneous structure, it is possible to use a phage cocktail composed of several bacteriophages showing bacteriolytic activity against various bacterial pathogens. Another alternative to enhance phage penetration through the matrix is debriding biofilm mechanically before phage treatment.
Another way to improve phage performance is to modify their genome or synthesize novel ones. Modified phages may have inserted gene coding for additional exopolysaccharide-degrading enzymes for better biofilm penetration. Bacteriostatic phages can be changed to suppress the DNA repair mechanism, or overexpression of sensitizing proteins, and to disrupt the cell-cell communication between the bacteria in the biofilm. Another target for phage engineering is to use phages as a modulator of antibiotic resistance or to make it possible to reach intracellular bacteria present in eukaryotic cells. Since sometimes bacterial lysis leads to the release of toxins and pro-inflammatory products, phages can be engineered to be toxic for bacteria but not lytic for their host.
Biofilms, phages and mammalian cells
Bacterial and phage composition in the intestine depends on diet and may drastically change during infection or other diseases. Increased or changed phage dsDNA levels were noticed during inflammatory bowel disease and type 1 diabetes in children. Phages may stably multiplicate in their host for weeks but not lead to the elimination of pathogens. Phages, as a component of the polymicrobial community, may cooperate with the mammalian immune system and actively eliminate bacteria from the lungs during infection.
Conversely, P. aeruginosa prophages can stabilize biofilm in the lungs by promoting attachment to lung mucus and restricting the dispersal of cells from the biofilm. Moreover, phages may decrease the pro-inflammatory response of the immune system and lead to chronic infection.
In another niche, lysogenic phages may modulate the number of vaginal lactobacilli during bacterial vaginosis. Phages are considered more effective against biofilm due to the production of polysaccharide depolymerases which loosen matrix structure and help antibiotics reach the bacteria cell surface. Another mode of action is the lysis of cells from exterior parts of biofilm which results in uncovering the deeper layers of cells and giving them access to nutrients and oxygen. This makes bacteria more metabolically active and more susceptible to an antibiotic. The effectiveness of such therapy also depends on the dosage of antibiotics and phages, time and order of administration, adsorption rate, burst size, latent period, and external physical factors such as pH and temperature.
To conclude
Biofilm eradication is an enlarging problem in medicine, agriculture, and the food industry. The Center for Disease Control and Prevention (CDC) estimates that even more than 65% of all chronic bacterial infections are caused by biofilm forms of pathogens.
Bacteria and other microorganisms prefer to organize themselves in multi-species communities. Such biofilms are difficult to cure using antibiotic therapy and to remove from abiotic surfaces. Due to the persistence of multi-species biofilms, alternative methods of their eradication are being developed. Bacteriophages are one of the solutions. Studies have been conducted using both wild-type and genetically modified or polyvalent phages. In addition, they can be successfully used in combination with antibiotics or other chemical molecules.
Microbial communities have mechanisms of protection from phages that affect phage’s ability to adsorb, penetrate, diffuse, and proliferate in biofilm. The ability of biofilm to resist phage invasion depends on its age, shape, structure, and morphology. Even though phages occurred to be effective to some point in biofilm-forming prevention and eradication of mature polymicrobial biofilm, some limitations of phage therapy must be overcome to achieve full effectiveness. The solution might be combined therapy based on phages mixed with antibiotics, nanoparticles and antimicrobial peptides or using phage-delivered enzymes with different properties that can give tailored results. Such a solution could be widely used in medicine to treat severe cases.
Read more : Gliźniewicz, M., Miłek, D., Olszewska, P., Czajkowski, A., Serwin, N., Cecerska-Heryć, E., … & Grygorcewicz, B. (2024). Advances in bacteriophage-mediated strategies for combating polymicrobial biofilms. Frontiers in Microbiology, 14, 1320345
Comments